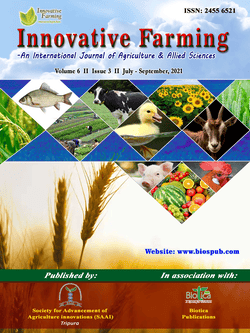
Plant Disease Responses to Climate Change
Jai P. Rai*
Institute of Agricultural Sciences, Banaras Hindu University, RG South Campus, Barkachha, Mirzapur, Uttar Pradesh (231 001), India
DOI: NIL
Keywords: Agriculture, Climate change, Crop losses, Pathogens, Plant diseases
Abstract
Environmental shift is a growing concern for communities related to agriculture across the world. The present paper looks into the impacts of shifts in climate on plant diseases. The three major constituents of any crop disease are the host (the plant) the pathogen inciting the disease, and the environmental factors. All these three elements are greatly affected by changes in climate that can lead to a surge in the occurrence and intensity of crop diseases. The paper highlights that escalating temperatures, changed patterns of rainfall and intense weather events can all create conditions that favour the growth and spread of phytopathogens. This can lead to substantial yield losses for farmers and put global food security at risk. The paper also discusses the economic consequences of crop diseases, noting that phytopathogens and insect pests are assessed to cause nearly US$ 220 billion in annual losses.
The author argues that a better understanding of how climate shifts affect the connection and correlation between pathogens, crop plants and the environment is necessary to develop strategies for mitigating crop disease risks. He calls for research into the molecular, epidemiological and ecological interactions between these factors. This research can inform the development of climate-durable farming procedures that can assist in ensuring food surety in a fluctuating climate.
Downloads
not found
Reference
Abdou Zayan, S., 2020. Impact of climate change on plant diseases and IPM strategies. In: Plant Diseases - Current Threats and Management Trends. (Ed.) Topolovec-Pintaric, S. IntechOpen. DOI: https://doi.org/10.5772/intechopen.87055.
Andersen, K.F., Madden, L.V., Paul, P.A., 2015. Fusarium head blight development and deoxynivalenol accumulation in wheat as influenced by post-anthesis moisture patterns. Phytopathology 105(2), 210-219. DOI: https://doi.org/10.1094/PHYTO-04-14-0104-R.
Barbeito, I., Brücker, R.L., Rixen, C., Bebi, P., 2013. Snow fungi-induced mortality of Pinus cembra at the alpine treeline: Evidence from plantations. Arctic, Antarctic and Alpine Research 45(4), 455-470. DOI: https://doi.org/10.1657/1938-4246-45.4.455.
Bidzinski, P., Ballini, E., Ducasse A., Michel C., Zuluaga, P., Genga, A., Chiozzotto, R., Morel, J.B., 2016. Transcriptional basis of drought-induced susceptibility to the rice blast fungus Magnaporthe oryzae. Frontiers in Plant Science 7, 1-13. DOI: https://doi.org/10.3389/fpls.2016.01558.
Brown, J.K.M., Hovmøller, M.S., 2002. Aerial dispersal of pathogens on the global and continental scales and its impact on plant disease. Science 297(5581), 537-541. DOI: https://doi.org/10.1126/science.1072678.
Burdon, J.J., Zhan, J., 2020. Climate change and disease in plant communities. PLoS Biology 18(11), e3000949. DOI: https://doi.org/10.1371/journal.pbio.3000949.
Castroverde, C.D.M., Dina, D., 2021. Temperature regulation of plant hormone signaling during stress and development. Journal of Experimental Botany 72(21), 7436-7458. DOI: https://doi.org/10.1093/jxb/erab257.
Chakraborty, S., Newton, A.C., 2011. Climate change, plant diseases and food security: An overview. Plant Pathology 60(1), 2-14. DOI: https://doi.org/10.1111/j.1365-3059.2010.02411.x.
Chaloner, T.M., Gurr, S.J., Bebber, D.P., 2021. Plant pathogen infection risk tracks global crop yields under climate change. Nature Climate Change 11, 710-715. DOI: https://doi.org/10.1038/s41558-021-01104-8.
Cheng, Y.T., Zhang, L., He, S.Y., 2019. Plant-microbe interactions facing environmental challenge. Cell Host & Microbe 26(2), 183-192. DOI: https://doi.org/10.1016/j.chom.2019.07.009.
Choi, H.K., Iandolino, A., da Silva, F.G., Cook, D.R., 2013. Water deficit modulates the response of Vitis vinifera to the Pierce’s disease pathogen Xylella fastidiosa. Molecular Plant-Microbe Interactions 26(6), 643-657. DOI: https://doi.org/10.1094/MPMI-09-12-0217-R.
Ciliberti, N., Fermaud, M., Roudet, J., Rossi, V., 2015. Environmental conditions affect Botrytis cinerea infection of mature grape berries more than the strain or transposon genotype. Phytopathology 105(8), 1090-1096. DOI: https://doi.org/10.1094/PHYTO-10-14-0264-R.
Coakley, S.M., Scherm, H., Chakraborty, S., 1999. Climate change and plant disease management. Annual Review of Phytopathology 37(1), 399-426. DOI: https://doi.org/10.1146/annurev.phyto.37.1.399.
Cohen, S.P., Liu, H., Argueso, C.T., Pereira, A., Vera Cruz, C., Verdier, V., Leach, J.E., 2017 RNA-seq analysis reveals insight into enhanced rice Xa7-mediated bacterial blight resistance at high temperature. PLoS ONE 12(11), e0187625. DOI: https://doi.org/10.1371/journal.pone.0187625.
Cohen, S.P., Leach, J.E., 2019. Abiotic and biotic stresses induce a core transcriptome response in rice. Scientific Reports 9, 6273. DOI: https://doi.org/10.1038/s41598-019-42731-8.
Cohen, S.P., Leach, J.E., 2020. High temperature-induced plant disease susceptibility: more than the sum of its parts. Current Opinion in Plant Biology 56, 235-241. DOI: https://doi.org/10.1016/j.pbi.2020.02.008.
Delgado-Baquerizo, M., Guerra, C.A., Cano-Díaz, C., Egidi, E., Wang, J., Eisenhauer, N., Singh, B.K., Maestre, F.T., 2020. The proportion of soil-borne pathogens increases with warming at the global scale. Nature Climate Change 10, 550-554. URL: https://doi.org/10.1038/s41558-020-0759-3.
Desaint, H., Aoun, N., Deslandes, L., Vailleau, F., Roux, F., Berthomé, R., 2021. Fight hard or die trying: When plants face pathogens under heat stress. New Phytologist 229(2), 712-734. DOI: https://doi.org/10.1111/nph.16965.
Desprez-Loustau, M.L., Marçais, B., Nageleisen, L.M., Piou, D., Vannini, A., 2006. Interactive effects of drought and pathogens in forest trees. Annals of Forest Science 63(6), 597-612. DOI: https://doi.org/10.1051/forest:2006040.
Dudney, J., Willing, C.E., Das, A.J., Latimer, A.M., Nesmith, J.C.B., Battles, J.J., 2021. Nonlinear shifts in infectious rust disease due to climate change. Nature Communications 12, 5102. DOI: https://doi.org/10.1038/s41467-021-25182-6.
Eastburn, D.M., Degennaro, M.M., Delucia, E.H., Dermody, O., Mcelrone, A.J., 2010. Elevated atmospheric carbon dioxide and ozone alter soybean diseases at SoyFACE. Global Change Biology 16(1), 320-330. DOI: https://doi.org/10.1111/j.1365-2486.2009.01978.x.
Fisher, M.C., Gurr, S.J., Cuomo, C.A., Blehert, D.S., Jin, H., Stukenbrock, E.H., Stajich, J.E., Kahmann, R., Boone, C., Denning, D.W., Gow, N.A.R., Klein, B.S., Kronstad, J.W., Sheppard, D.C., Taylor, J.W., Wright, G.D., Heitman, J., Casadevall, A., Cowen, L.E., 2020. Threats posed by the fungal kingdom to humans, wildlife and agriculture. mBio 11(3), e00449-20. DOI: https://doi.org/10.1128/mbio.00449-20.
Fones, H.N., Bebber, D.P., Chaloner, T.M., Kay, W.T., Steinberg, G., Gurr, S.J., 2020. Threats to global food security from emerging fungal and oomycete crop pathogens. Nature Food 1, 332-342. DOI: https://doi.org/10.1038/s43016-020-0075-0.
Goellner, K., Loehrer, M., Langenbach, C., Conrath, U., Koch, E., Schaffrath, U., 2010. Phakopsora pachyrhizi, the causal agent of Asian soybean rust. Molecular Plant Pathology 11(2), 169-177. DOI: https://doi.org/10.1111/j.1364-3703.2009.00589.x.
Hossain, M., Veneklaas, E.J., Hardy, G.E., Poot, P., 2019. Tree host-pathogen interactions as influenced by drought timing: Linking physiological performance, biochemical defence and disease severity. Tree Physiology 39(1), 6-18. DOI: https://doi.org/10.1093/treephys/tpy113.
Islam, M.T., Kim, K.H., Choi, J., 2019. Wheat blast in Bangladesh: The current situation and future impacts. The Plant Pathology Journal 35(1), 1-10. DOI: https://doi.org/10.5423/PPJ.RW.08.2018.0168.
Johansen, T.J., Dees, M.W., Hermansen, A., 2015. High soil moisture reduces common scab caused by Streptomyces turgidiscabies and Streptomyces europaei scabiei in potato. Acta Agriculturae Scandinavica, Section B - Soil & Plant Science 65(3), 193-198. DOI: https://doi.org/10.1080/09064710.2014.988641.
Juroszek, P., von Tiedemann, A., 2015. Linking plant disease models to climate change scenarios to project future risks of crop diseases: A review. Journal of Plant Diseases and Protection 122, 3-15. DOI: https://doi.org/10.1007/BF03356525.
Khan, M.R., Rizvi, T.F., 2020. Effect of elevated levels of CO2 on powdery mildew development in five cucurbit species. Scientific Reports 10, 4986. DOI: https://doi.org/10.1038/s41598-020-61790-w.
Karnosky, D.F., Percy, K.E., Xiang, B., Callan, B., Noormets, A., Mankovska, B., Hopkin, A., Sober, J., Jones, W., Dickson, R.E., Isebrands, J.G., 2002. Interacting elevated CO2 and tropospheric O3 predisposes aspen (Populus tremuloides Michx.) to infection by rust (Melampsora medusae f. sp. tremuloidae). Global Change Biology 8(4), 329-338. DOI: https://doi.org/10.1046/j.1354-1013.2002.00479.x.
Ma, L., Qiao, J., Kong, X., Zou, Y., Xu, X., Chen, X., Hu, X., 2015. Effect of low temperature and wheat winter-hardiness on survival of Puccinia striiformis f. sp. tritici under controlled conditions. PLoS ONE 10(6), e0130691. DOI: https://doi.org/10.1371/journal.pone.0130691.
Mamo, B.E., Eriksen, R.L., Adhikari, N.D., Hayes, R.J., Mou, B., Simko, I., 2021. Epidemiological characterization of lettuce drop (Sclerotinia spp.) and biophysical features of the host identify soft stem as a susceptibility factor. PhytoFrontiers 1(3), 182-204. DOI: https://doi.org/10.1094/PHYTOFR-12-20-0040-R.
Mcelrone, A.J., Reid, C.D., Hoye, K.A., Hart, E., Jackson, R.B., 2005. Elevated CO2 reduces disease incidence and severity of a red maple fungal pathogen via changes in host physiology and leaf chemistry. Global Change Biology 11(10), 1828-1836. DOI: https://doi.org/10.1111/j.1365-2486.2005.001015.x.
Muluneh, M.G., 2021. Impact of climate change on biodiversity and food security: a global perspective - a review article. Agriculture and Food Security 10, 36. DOI: https://doi.org/10.1186/s40066-021-00318-5.
Newbery, F., Qi, A., Fitt, B.D.L., 2016. Modelling impacts of climate change on arable crop diseases: Progress, challenges and applications. Current Opinion in Plant Biology 32, 101-109. DOI: https://doi.org/10.1016/j.pbi.2016.07.002.
Oliva, J., Stenlid, J., Martinez-Vilalta, J., 2014. The effect of fungal pathogens on the water and carbon economy of trees: Implications for drought-induced mortality. New Phytologist 203(4), 1028-1035. DOI: https://doi.org/10.1111/nph.12857.
Parikka, P., Hakala, K., Tiilikkala, K., 2012. Expected shifts in Fusarium species’ composition on cereal grain in Northern Europe due to climatic change. Food Additives & Contaminants: Part A 29(10), 1543-1555. DOI: https://doi.org/10.1080/19440049.2012.680613.
Qiu, J., Dong, F., Yu, M., Xu, J., Shi, J., 2016. Effect of preceding crop on Fusarium species and mycotoxin contamination of wheat grains. Journal of the Science of Food and Agriculture 96(13), 4536-4541. DOI: https://doi.org/10.1002/jsfa.7670.
Rai, A., Irulappan, V., Senthil-Kumar, M., 2022. Dry root rot of chickpea: A disease favored by drought. Plant Disease 106(2), 346-356. DOI: https://doi.org/10.1094/PDIS-07-21-1410-FE.
Rigg, J.L., McDougall, K.L., Liew, E.C.Y., 2018. Susceptibility of nine alpine species to the root rot pathogens Phytophthora cinnamomi and P. cambivora. Australasian Plant Pathology 47, 351-356. DOI: https://doi.org/10.1007/s13313-018-0564-x.
Ristaino, J.B., Anderson, P.K., Bebber, D.P., Brauman, K.A., Cunniffe, N.J., Fedoroff, N.V., Finegold, C., Garrett, K.A., Gilligan, C.A., Jones, C.M., Martin, M.D., MacDonald, G.K., Neenan, P., Records, A., Schmale, D.G., Tateosian, L., Wei, Q., 2021. The persistent threat of emerging plant disease pandemics to global food security. PNAS 118(23), e2022239118. DOI: https://doi.org/10.1073/pnas.2022239118.
Rohr, J.R., Barrett, C.B., Civitello, D.J., Craft, M.E., Delius, B., DeLeo, G.A., Hudson, P.J., Jouanard, N., Nguyen, K.H., Ostfeld, R.S., Remais, J.V., Riveau, G., Sokolow, S.H., Tilman, D., 2019. Emerging human infectious diseases and the links to global food production. Nature Sustainability 2, 445-456. DOI: https://doi.org/10.1038/s41893-019-0293-3.
Ryu, M., Mishra, R.C., Jeon, J., Lee, S.K., Bae, H., 2018. Drought-induced susceptibility for Cenangium ferruginosum leads to progression of Cenangium-dieback disease in Pinus koraiensis. Scientific Reports 8, 16368. DOI: https://doi.org/10.1038/s41598-018-34318-6.
Sewelam, N., El-Shetehy, M., Mauch, F., Maurino, V.G., 2021. Combined abiotic stresses repress defense and cell wall metabolic genes and render plants more susceptible to pathogen infection. Plants 10(9), 1946. DOI: https://doi.org/10.3390/plants10091946.
Sikes, B.A., Bufford, J.L., Hulme, P.E., Cooper, J.A., Johnston, P.R., Duncan, R.P., 2018. Import volumes and biosecurity interventions shape the arrival rate of fungal pathogens. PLoS Biology 16(5), e2006025. DOI: https://doi.org/10.1371/journal.pbio.2006025.
Sparks, A.H., Forbes, G.A., Hijmans, R.J., Garrett, K.A., 2014. Climate change may have limited effect on global risk of potato late blight. Global Change Biology 20(12), 3621-3631. DOI: https://doi.org/10.1111/gcb.12587.
Tada, T., Tanaka, C., Katsube-Tanaka, T., Shiraiwa, T., 2021. Effects of wounding and relative humidity on the incidence of Phytophthora root and stem rot in soybean seedlings. Physiological and Molecular Plant Pathology 116, 101737. DOI: https://doi.org/10.1016/j.pmpp.2021.101737.
Teshome, D.T., Zharare, G.E., Naidoo, S., 2020. The threat of the combined effect of biotic and abiotic stress factors in forestry under a changing climate. Frontiers in Plant Science 11, 601009. DOI: https://doi.org/10.3389/fpls.2020.601009.
Thompson, S.E., Levin, S., Rodriguez-Iturbe, I., 2014. Rainfall and temperatures changes have confounding impacts on Phytophthora cinnamomi occurrence risk in the southwestern USA under climate change scenarios. Global Change Biology 20(4), 1299-1312. https://doi.org/10.1111/gcb.12463.
Toniutti, L., Breitler, J.C., Etienne, H., Campa, C., Doulbeau, S., Urban, L., Lambot, C., Pinilla, J.H., Bertrand, B., 2017. Influence of environmental conditions and genetic background of Arabica coffee (C. arabica L.) on leaf rust (Hemileia vastatrix) pathogenesis. Frontiers in Plant Science 8, 2025. DOI: https://doi.org/10.3389/fpls.2017.02025.
Trębicki, P., Vandegeer, R.K., Bosque-Pérez, N.A., Powell, K.S., Dader, B., Freeman, A.J., Yen, A.L., Fitzgerald, G.J., Luck, J.E., 2016. Virus infection mediates the effects of elevated CO2 on plants and vectors. Scientific Reports 6, 22785. DOI: https://doi.org/10.1038/srep22785.
Trivedi, P., Leach, J.E., Tringe, S.G., Sa, T., Singh, B.K., 2020. Plant-microbiome interactions: From community assembly to plant health. Nature Reviews Microbiology 18(11), 607-621. DOI: https://doi.org/10.1038/s41579-020-0412-1.
Trumbore, S., Brando, P., Hartmann, H., 2015. Forest health and global change. Science 349(6250), 814-818. DOI: https://doi.org/10.1126/science.aac6759.
van Dijk, M., Morley, T., Rau, M.L., Saghai, Y., 2021. A meta-analysis of projected global food demand and population at risk of hunger for the period 2010-2050. Nature Food 2, 494-501. DOI: https://doi.org/10.1038/s43016-021-00322-9.
Vary, Z., Mullins, E., McElwain, J.C., Doohan, F.M., 2015. The severity of wheat diseases increases when plants and pathogens are acclimatized to elevated carbon dioxide. Global Change Biology 21(7), 2661-2669. DOI: https://doi.org/10.1111/gcb.12899.
Velasquez, A.C., Castroverde, C.D.M., He, S.Y., 2018. Plant-pathogen warfare under changing climate conditions. Current Biology 28(10), R619-R634. DOI: https://doi.org/10.1016/j.cub.2018.03.054.
Wakelin, S., Gómez-Gallego, M., Jones, E., Smaill, S., Lear, G., Lambie, S., 2018. Climate change induced drought impacts on plant diseases in New Zealand. Australasian Plant Pathology 47, 101-114. DOI: https://doi.org/10.1007/s13313-018-0541-4.
Walter, S., Ali, S., Kemen, E., Nazari, K., Bahri, B.A., Enjalbert, J., Hansen, J.G., Brown, J.K., Sicheritz-Pontén, T., Jones, J., de Vallavieille-Pope, C., Hovmøller, M.S., Justesen, A.F., 2016. Molecular markers for tracking the origin and worldwide distribution of invasive strains of Puccinia striiformis. Ecology and Evolution 6(9), 2790-2804. DOI: https://doi.org/10.1002/ece3.2069.
Webb, K.M., Oña, I., Bai, J., Garrett, K.A., Mew, T., Vera Cruz, C.M., Leach, J.E., 2010. A benefit of high temperature: Increased effectiveness of a rice bacterial blight disease resistance gene. The New Phytologist 185(2), 568-576. DOI: https://doi.org/10.1111/j.1469-8137.2009.03076.x.
Xin, X.F., Nomura, K., Aung, K., Velásquez, A.C., Yao, J., Boutrot, F., Chang, J.H., Zipfel, C., He, S.Y., 2016. Bacteria establish an aqueous living space in plants crucial for virulence. Nature 539(7630), 524-529. DOI: https://doi.org/10.1038/nature20166.
Zhan, J., Ericson, L., Burdon, J.J., 2018. Climate change accelerates local disease extinction rates in a long-term wild host-pathogen association. Global Change Biology 24(8), 3526-3536. DOI: https://doi.org/10.1111/gcb.14111.
Zhou, Y., Van Leeuwen, S.K., Pieterse, C.M.J., Bakker, P.A.H.M., Van Wees, S.C.M., 2019. Effect of atmospheric CO2 on plant defense against leaf and root pathogens of Arabidopsis. European Journal of Plant Pathology 154, 31-42. DOI: https://doi.org/10.1007/s10658-019-01706-1.